Guangdong Provincial Key Laboratory of Infectious Diseases and Molecular Immunopathology, Shantou University Medical College, Shantou 515041, China
Geometry Cell Biology Research Center, Dongguan 523808, China
The Center for Reproductive Medicine, Shantou University Medical College, Shantou 515041, China
Author to whom correspondence should be addressed.
Abstract
Nonalcoholic fatty liver disease (NAFLD) is a chronic liver disorder characterized by an enhanced accumulation of lipids, which affects around 40% of the world’s population. The T. fuciformis fungus possesses immunomodulatory activity and other beneficial properties that may alleviate steatosis through a different mechanism. The present study was designed to evaluate the effect T. fuciformis crude polysaccharides (TFCP) on inflammatory and lipid metabolism gene expression, oxidative stress, and lipid profile. Mice were divided into groups receiving (a) a normal chow diet (NCD), (b) a methionine–choline-deficient (MCD) diet, and (c) a MCD diet with TFCP. Liver histopathology was performed, and the hepatic gene expression levels were estimated using qRT-PCR. The lipid profiles, ALT, AST, and efficient oxidative enzymes were analyzed using ELISA. The TFCP administration in the MCD-fed mice suppressed hepatic lipid accumulation, lipid metabolism-associated genes (HMGCR, FABP, SREBP, ACC, and FAS), and inflammation-associated genes (IL-1β, TLR4, TNF-α, and IL-6) whilst enhancing the expression of HNF4α genes. TFCP mitigated against oxidative stress and normalized healthy lipid profiles. These results highlighted that TFCP prevents NAFLD through the inhibition of oxidative stress and inflammation, suggesting TFCP would potentially be an effective therapeutic agent against
1. Introduction
Nonalcoholic fatty liver disease (NAFLD) is a severe hepatic disorder causing a huge overall burden of liver diseases [1]. NAFLD may be defined as the umbrella term covering a range of abnormalities, from NAFLD to nonalcoholic steatohepatitis (NASH), which may progress to fibrosis and cirrhosis [1]. NAFLD is closely associated with metabolic syndrome. The abnormalities that develop in NAFLD patients include (i) abdominal obesity, (ii) high blood pressure, (iii) high blood glucose, (iv) high serum triglycerides, and (v) a low abundance of serum high-density lipoprotein cholesterol (HDL-C), which was reported to contribute significantly to the disease [2]. The ethnicity of the patients influences NAFLD development [3]. In in vivo models, the methionine–choline-deficient (MCD) diet was reported to induce steatohepatitis; however, it does not influence body weight gain and obesity [4]. Additionally, a high occurrence of NAFLD was observed in obese and diabetic patients, while non-diabetic and lean NAFLD patients were also reported to a lesser extent [5].
To date, a huge amount of research has evidenced the crucial role of toll-like receptor 4 (TLR4) and dysbiosis in NAFLD development [5]. Indeed, gut microbiota (GM) have been found to extend their axes bi-directionally (i.e., endocrine and immunological pathways) with numerous extra-intestinal organs, such as the brain, kidneys, and bone [4]. Nevertheless, this gut–liver axis plays an essential role during the progression of chronic liver diseases, such as hepatitis B and C, NAFLD, NASH, and hepatocellular carcinoma (HCC) [6]. GM-induced dysbiosis enhances the hepatic TLR4 expression and pro-inflammatory cytokine levels in NAFLD and NASH patients by disrupting the intestine’s inner lining and prompting the lipopolysaccharide (LPS) translocation in TLR4 signaling activation [7]. Thus, LPS–TLR4 pathways in NAFLD pathogenesis could be marked as a significant cause of NASH [5].
T. fuciformis is a well-known edible mushroom and was reportedly used in ancient Chinese society as a traditional medicine [8,9]. The T. fuciformis fungus possesses antitumor, anti-inflammatory, and immunomodulatory properties [10]. T. fuciformis administration has been reported to significantly improve the serum IL-2, IL-12, INF-γ, and IgG levels in cyclophosphamide-induced immunosuppressed mice and show immunomodulatory abilities [8]. Additionally, the T. fuciformis fungus may alleviate colonic tissue damage in colitic mice by shortening the length of the colon and lowering the concentration of D-lactate [10].
The hepatocyte nuclear factor 4α (HNF4α, NR2A1) is a member of the superfamily of nuclear hormone receptors that are expressed in the liver, kidney, pancreatic islets, and gut and is engaged in regulating the gene expression involved in fatty acid, cholesterol, glucose, and urea synthesis [11]. HNF4α plays a significant role in lowering inflammation, and the reduced expression of HNF4α was observed in alcoholic and nonalcoholic steatohepatitis patients [12]. In contrast, the overexpression of HNF4α has been shown to promote a substantial anti-inflammatory effect in mesenchymal stem cells (MSC) through a nuclear factor kappa B (NF-κB)-dependent pathway [13].
Considering the above background, this study was designed to investigate the effects of TFCP on intrinsic immunogenic and metabolic activities in methionine–choline-deficient (MCD) mice with diet-induced NAFLD. Here, we studied the impact of TFCP on the serum parameters, including lipid profile, antioxidants, liver enzymes (ALT and AST), and the gene expression of inflammatory cytokines (IL-1β, HNF4α, TLR4, TNF-α, and IL-6) and lipid metabolism-associated genes (HMGCR, FABP, SREBP, ACC, and FAS) in the MCD-diet-induced NAFLD mice. Our results indicated that TFCP could help to alleviate NAFLD by suppressing inflammation.
2. Materials and Methods
2.1. TFCP Preparation and Characterization
T. fuciformis crude polysaccharides (TFCP), provided by Dr Xiaofei Xu, were obtained from the fruit body of T. fuciformis by boiling water extraction. Dry T. fuciformis fruiting bodies were collected from Gutian, Fujian province, China. The T. fuciformis fruiting bodies were crushed and sifted through a 40 mesh prior to use. TFCP was extracted using the hot water extraction method (1:60 w/v) at 95 °C for 6 h. Then, the solution was filtered and concentrated to quarter of its original weight under vacuum conditions at 70 °C. Deproteination was performed by adjusting the pH of the solution to 4.0 using citric acid and it was then kept at 4 °C for 2 days by isoelectric precipitation. Collecting supernatant and three equivalent volumes of 95% ethanol were added to the precipitate polysaccharides at 4 °C overnight. The TFCP fraction was obtained by centrifugation and lyophilization. The TFCP powder was white in color. The peak molecular weight of the TFCP was about 1100 kDa, as measured using high-performance gel permeation chromatography (HPGPC). The saccharide content of the TFCP was 82%, as detected by phenol sulfuric acid analysis with mannose as a standard. Protein content was measured by the Bradford method. The protein content was 2.4% in the TFCP fraction. Fourier transform infrared (FT-IR) spectroscopy of the TFCP samples was measured to be in the range of 4000–400 cm−1 by the KBr disk method using an FT-IR spectrometer (Nicolet IS50-Nicolet Continuum, Thermo Fisher Scientific). OMNIC software was used for the spectroscopy analysis. As shown in following figure, the characteristic peaks of FT-IR spectra of the TFCP fraction at approximately 3412, 2935, 1721, 1606, 1424, 1135, 1071, 915, and 803 cm−1 are presented. The absorbance at 3412 and 2935 cm−1 are due to the stretching of O–H and -CH2 groups, respectively. The peaks at 1721 and 1606 cm−1 demonstrate the presence of the uronic acid group. High absorbance in the region of 1200–1000 cm−1 is typical for the polysaccharides molecule through the stretching of C–O, C–C, and C–OH. The peak at 915 cm−1 is due to the presence of D-glucopyranosyl and the peak at 803 cm−1 suggests the presence of α-glycosidic bonds in the molecular structure of the TFCP.
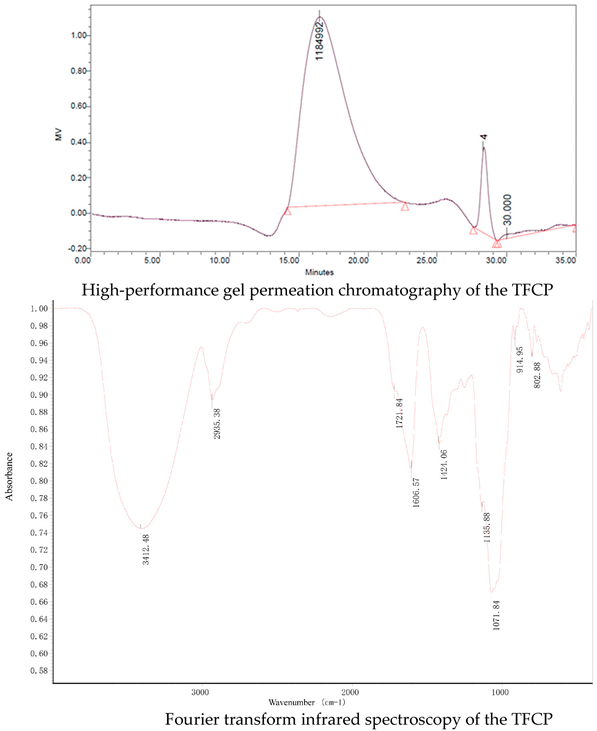
2.2. Animal Experiments
For the in vivo experiments, specific pathogen-free (SPF) male Kunming mice (18–22 g) were housed in a controlled environment (23 °C, 12 h daylight cycle, lights off at 18:00 h, and relative humidity was 40–50%). Notably, the Institutional Animal Care and Use Committee of Shantou University Medical College provided the desired approvals and supervision for the animal experiments according to the National Research Council Guide for Care and Use of Laboratory Animals.
Following the standard protocols, all mice were kept for seven days with free access to water and a standard chow diet to acclimatize before treatments. The mice were then divided randomly into three different groups based on diet and/or treatment: the NCD control group (n = 5); the methionine–choline-deficient (MCD) diet group (n = 5); and the intervention group (MCD + TFCP) (n = 5). Mice from the control group were fed the standard diet with free access to water. The MCD diet contained 40% sucrose and 10% fat as ingredients but lacked methionine and choline, which are necessary for hepatic mitochondrial β-oxidation [14]. The MCD and MCD + TFCP groups were fed the methionine–choline-deficient (MCD) diet. The intervention group underwent daily intragastric administration of TFCP (200 mg per kg body weight per day). In contrast, the MCD group received the same amount of normal saline once per day for six weeks [10].
After six weeks of the study, the mice were fasted for 12 h and then we collected blood and tissue samples. All animals were euthanized using pentobarbital sodium.
2.3. Collection of Liver Tissue and Their Histopathological Analysis
Mice (treated and untreated) were considered for dissection to collect hepatic tissues for histopathological analysis. Liver samples were excised immediately after sacrifice using a scalpel and placed into appropriate tubes for the histopathologic examinations. For histological analysis, the liver tissues were fixed in 4% paraformaldehyde for 4 h. Paraformaldehyde-fixed paraffin sections of the liver were stained with hematoxylin–eosin (HE) for pathological analysis. The images were captured by an optical microscope (Leica DMI3000B, USA).
2.4. Serum and Blood Measurements
Alanine aminotransferase (ALT), aspartate aminotransferase (AST), and high-density lipoprotein (HDL-C) in the serum were quantitated using an automated analyzer (Sysmex CHEMIX-180, Japan). The concentrations of serum triglycerides (TG) and total cholesterol (TC) were separately quantitated using triglyceride and cholesterol assay kits (Applygen Technologies Inc., Beijing, China) according to the manufacturer’s instructions. Additionally, the oxidative stress was elucidated by measuring the malondialdehyde (MDA) and superoxide dismutase (SOD) enzymes in the serum samples following ELISA-based standard protocols.
2.5. Real-Time Quantitative Polymerase Chain Reaction
Total RNA was extracted from the liver using TRIzol (D9108B, Takara, Dalian, China) and reverse-transcribed into cDNA using PrimeScript RT master mix (RR036A, Takara, Dalian, China). Real-time quantitative polymerase chain reaction (qRT-PCR) was performed with Applied Biosystems 7500 real-time PCR system using the SYBR Premix Ex Taq (Tli RNase H Plus) (RR420A, Takara, Dalian, China). The primers of the target genes were synthesized by Sangon Biotech (Shanghai, China). Glyceraldehyde 3-phosphate dehydrogenase (GAPDH) (B661304, Sangon Biotech) was used as the internal control.
2.6. Statistical Analysis
Data were expressed as the mean ± SEM. Statistical analyses were performed using the student t-test in IBM SPSS Statistics 20. p < 0.05 was considered statistically significant. Drawn figures were created using GraphPad Prism 8.